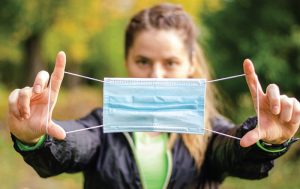
Manufacturing innovations will provide more reliable, affordable and adaptable protection in the face of the next pandemic.
By Behnam Pourdeyhimi, Technical Editor; Simon Schick; and Robert Groten
The World Health Organization (WHO) recently released the findings of its investigation into what went right and wrong as the world was faced with the coronavirus pandemic. An 86-page report noted that there were “weak links at every point in the chain of preparedness and response.” A 13-member panel appointed by the WHO called the COVID-19 pandemic a “preventable disaster.”
Some of the recommendations resulting from the investigation included:
- Creating an improved system for disease surveillance and alerts, at a speed that can combat viruses like the one that causes COVID-19, along with authority for WHO to publish information and to dispatch expert missions immediately.
- The ability to produce vaccines, diagnostics, therapeutics and supplies and secure their rapid and equitable delivery as essential global common goods.
- Production of and access to COVID-19 tests and therapeutics, including oxygen, should be scaled up urgently in low- and middle-income countries.
Vice President Kamala Harris and U.S. Ambassador Linda Thomas-Greenfield recently opened a dialogue on pandemic preparedness aimed at learning the lessons learned from COVID-19, which was cohosted by Argentina, Norway, Japan, South Africa and the United States.
“The takeaway from this past year is clear: the world barely withstood this pandemic,” said Thomas-Greenfield addressing the global group comprised of political, public health and non-governmental organization leaders. “We must be ready for the next. We cannot continue to under-invest — after outbreaks, epidemics, and now a pandemic — in our capacity to prevent, detect, and respond to infectious disease threats.”
Note that the WHO does not specifically address the challenges with personal protective equipment (PPE), but focuses on challenges with therapeutics, among other solutions. The second bullet point above is a key point — having the ability to produce what is needed quickly and deliver these supplies globally and equitably.
When it comes to such products as face masks for example, it is difficult to produce them quickly and distribute them equitably to low- and middle-income countries. A paradigm shift is needed, and cloth masks are not the solution
Background
Protective mouth and nose coverage can be traced back to the beginning of the 20th century. In 1897, Carl Friedrich Flügge discussed the possibility of droplet infections originated by coughing and, interestingly, first identified the importance of social distancing1,2. The airborne transmission of cholera, plague, and cerebrospinal meningitis were discussed and the importance of wearing a “mouth bandage” — a single layer of cotton gauze — was recognized1. Cotton-based face masks were subsequently widely used during the Spanish flu pandemic of 1918-1920.
In contrast to this simple cloth-based technology, current respirators and face masks center on two key technologies: fine, meltblown fibers; and an electrostatic charge. Meltblowing itself extends back to 1941 when the process was first invented3. It was immediately recognized that meltblowing can produce very fine fibers relative to traditional fiber melt spinning4. Meltblowing is a very economical, large-scale process that creates fiber diameters that range from sub-micron to a few microns.
Electrostatic charge enhances the particle interception efficiency of such fibers while producing a significantly lower overall pressure drop — a measure of the resistance that the air meets as it flows through the mask, which should be low enough that the effort required to breath is as normal as possible.
Other critical technologies focused on ensuring that the charge density was initially high and, more importantly, stable. The first “modern” mask was introduced in 19675 while the first respirator was patented in 1976. A critical development was the application of electrostatic charge to meltblown webs in 19806. Key patents leading to the current widely used N95 respirators soon followed. The first such patent was issued to Saint Paul, Minn.-based 3M Co. in 1980 when a meltblown structure was charged to form a high efficiency electrostatic filter7. In 1985, 3M developed the first modern, cup-shaped molded respirator8.
Ideal Filtration
Aerosols are a suspension of solid or liquid particles in a gas ranging in size from 2 nanometers (nm) to 100 micrometers. Bioaerosols are aerosols of biological origin, including viruses, bacteria and fungi. An ideal filter removes only the unwanted aerosol particles from the air and does it without creating a large pressure drop. There are four primary aerosol filtration mechanisms9 (see Figure 1).
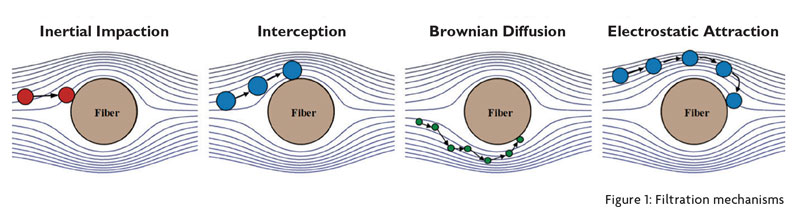
The air stream bends as it moves around the fibers. Large particles are trapped by inertial impaction. Large particles have a high probability of impacting with a fiber because inertia causes them to deviate from the streamline. Inertial impaction is for large micro particles and becomes important at high and medium velocities. Very small particles also have a high probability of hitting a fiber due to Brownian motion. Brownian motion comes about as the particles collide with gas (air) molecules that are much smaller than the particles. This leads to a chaotic movement that is disordered and abrupt, which leads to diffusion. The capture mechanism for particles less than 100 nm is mainly due to diffusion.
However, larger sized particles — from approximately 100 nm to 400 nm with roughly 300 nm representing the most challenging particle size to capture — normally follow the airflow streamlines and are the most difficult to capture. The capture mechanism is due to interception as the particles may be intercepted by a fiber. This is where the electrostatic attraction becomes important. Oppositely charged particles are attracted to a charged fiber. This collection mechanism does not favor a certain particle size particularly but is most significant for the particles most difficult to capture.
Fiber size becomes critical for the interception capture mechanism. If the fibers are of the same size or smaller, they have a tendency to be more effective in intercepting the particles. This, together with high electrostatic attraction, leads to high filtration collection efficiency in today’s respirators and medical masks that reply upon charged meltblown structures.
Cloth masks produced from regular textile fibers employ much larger fibers — greater than 15 microns in most cases — lack electrostatic charge and the structures create large gaps or holes. These gaps lead to localized areas that have a much lower pressure drop and therefore the air stream will flow naturally to these areas, carrying the aerosol particles through the structure. These masks offer almost no respiratory protection. They are useful however in reducing the number of expelled particles from the wearer, which may result in reducing the spread of a virus even though they may not offer much protection to the wearer. Thus, cloth masks are useful as a means of source control for individual wearers by reducing the number of expelled droplets and aerosols from the wearer’s nose and mouth into the air10.
Challenges During COVID-19
Mask filters are almost exclusively made from electrostatically charged polypropylene (PP) meltblown (MB) nonwovens. MB filters have fibers in the range of 0.5 to 5 microns in size and are therefore, fragile and must be protected by layers of spunbond (SB) PP made up of larger fibers — 15 to 25 microns — which provide mechanical protection for the MB filter layer.
The overall supply chain consists of the following:
- MB fabric manufacturers;
- SB fabric manufacturers;
- Mask converters who assemble the MB and SB fabrics into masks.
The dual challenge faced in the United States and globally during the COVID-19 pandemic was the limited production of MB fabric and the lack of infrastructure needed to convert the fabrics into masks.
MB and SB production facilities are always custom built, are large and expensive and require significant infrastructure. The lead time for setting up new capabilities is typically greater than 10 months at a cost of more than $10 million for a small, 1.6-meter-wide meltblowing machine. Therefore, even in high-income regions in North America and the European Union, plants could not be deployed any faster. In other regions of the world, it would be impossible to ramp up quickly, and this is why there needs to be a paradigm shift. In contrast, mask conversion is more readily available, is relatively inexpensive and does not require special infrastructure.
MB machines are a lot more expensive that the sum of their components. Engineering know-how and in some cases, intellectual property, create barriers to entry. Meltblowing, for example, is rather simple as a process. The key to the technology is the die tip that controls the uniformity of the fibers produced. The rest of the components are off-the-shelf. However, the nonwoven industry is built around large volumes, high speed and low production costs, and automation. This is why the nonwoven industry continues to lead the world in productivity and innovation.
What is needed for such products as face masks and respirators is a different solution. The mask conversion is also a fully automated process, and the width requirements range from 19.5 centimeters (cm) to 32 cm at most. Note that mask converting does not require special infrastructure such as high bay space, for example. If a MB machine was designed that is only say 40 cm wide, the process will still be fast enough to supply many mask converting machines while the costs will be low, and no special infrastructure is needed. Take for example, the Biax Fiber Film pilot line which measures 15 inches (38 cm) in width. With a few minor changes, this can become a super mask filter production machine that can also be collocated with the mask converting equipment. There are other examples of such smaller footprint lines that are primarily used as pilot lines. Such designs can be replicated and reproduced easily as needed.
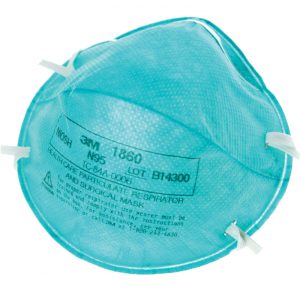
United States for mask production is
for the three-layer surgical mask, not
the N95 mask. (Brian McGowan/Unsplash)
Lack Of Know-How, Equipment Availability
Another major challenge during the pandemic was lack of know-how. Meltblown structures require additives as charge enhancers and charge stabilizers. While some masterbatches are commercially available, some work and some do not. Also, while meltblown machinery is fully automated, the resulting fabric is impacted by process variables and therefore, a trial-and-error approach is required to establish the process conditions that lead to the desired performance characteristics. While there were companies in North America producing other meltblown products, switching to filter media was not a simple task.
Another challenge faced in North America was that no one was producing mask converting machinery before the pandemic. This was not because of a lack of expertise or capabilities, but rather driven by simple economics. Asian-made machines sold for as little as $30,000 to as much as $120,000 and could be delivered in a few weeks. The cost of the components in North America would be higher than the finished product and given that the machines look similar and operate similarly, the tendency has been to lean toward Asian-made machinery. Lack of technical support, an inadequate supply of parts, and the unreliability of the machinery, often led to delays and much higher production costs.
Some sixteen months later, the situation has changed. There are a few new installations for meltblowing and according to the Cary, N.C.-based Association of the Nonwoven Fabrics Industry (INDA), there are more than 70 companies who have invested in mask converting equipment since the beginning of the pandemic. Much of the new machinery, however, is for the production of the classical three-layer surgical mask. With cheap Asian surgical masks flooding the market over the past six months, it is questionable whether many of the companies that made investments will be able to compete. This, in turn, will lead to challenges for the MB capacity installed.
There are far fewer N95 machines installed. In addition, medical masks and respirators are regulated by the National Institute for Occupational Safety and Health (NIOSH), the U.S. Food and Drug Administration (FDA) and the Occupational Safety and Health Administration (OSHA), and another barrier to entering the market today is the long lead time required for certification. Many companies have been waiting for certification for many months and the queue is not getting shorter. Certification also requires the development of a quality management system such as International Organization for Standardization (ISO) or good manufacturing practice (GMP) certification — additional barriers to market entry.
What About The Next Pandemic?
It is not a question of if, but when, another global pandemic happens. In the absence of policies that encourage domestic manufacturing of PPE and other critical products, the shortage situation in the United States likely will be no different. In the absence of democratized manufacturing, low-income countries will continue to be at a disadvantage.
The status quo of PPE manufacturing is not advanced enough to shield the United States from the next viral outbreak definitively. Further manufacturing innovations are the path forward to more reliable, affordable, adaptable protection. Cloth masks will not be the solution, and will be regulated perhaps using the new ASTM 3502 or its equivalent.
The Nonwovens Institute, Raleigh, N.C., and its partners are developing manufacturing strategies and educational programs to help establish definitive minimum standards for manufacturing that would be scalable and easily reproducible.
References:
1 Flügge, C. Ueber Luftinfection. Zeitschr. f. Hygiene. 25, 179–224 (1897). https://doi.org/10.1007/BF02220473
2 C. Flügge, The spread of phthisis through dusty sputum and through droplets that are sprayed out when coughing, Journal of Hygiene and Infectious Diseases volume 30 , pages107 – 124 ( 1899 )
3 JG McCulloch, The history of the development of melt blowing technology International Nonwovens Journal, 1999
4 Wente, V.A. , Naval Research Laboratory, Report 4364, Manufacture of Superfine Fibers. May 25, 1954.
5 U.S. Patent 3,333,585
6 U.S. Patent 3,971,373A
7 U.S. Patent 4,215,682A
8 U.S. Patent 4,536,440A
9 Pourdeyhimi, Schick and Groten, The Filtration Efficiency of Single-Layer Textiles for Respiratory protection, The Journal of Science and Medicine, Vol 3 No 2 (2021)
10 Pourdeyhimi, Schick and Groten, Respiratory Effectiveness of Cloth Masks, The Journal of Science and Medicine, Vol 2 No 4 (2021)
Editor’s Note: Dr. Behnam Pourdeyhimi is associate dean for Industry Research and Extension, William A. Klopman Distinguished Professor of Materials, professor of Chemical and Biomolecular Engineering and Biomedical Engineering, and executive director, The Nonwovens Institute (NWI), Wilson College of Textiles, North Carolina State University, Raleigh, N.C.; Simon Schick was a masters student at NWI and NC State; and Robert Groten is a professor with Germany-based Hochschule Niederrhein University of Applied Sciences, Germany, and an adjunct professor at NC State.
July/August 2021