By Marie-Isabel Popzyk, Viktor Reimer and Thomas Gries
Introduction And Motivation Of The Project GreenBraid
Bio composites — natural fiber reinforced composites — for structural applications have proven their material capabilities on the various levels of aggregation — the micro (fibers), meso (textile) and macro (part) level. Bio composites are on their way to beat glass-based composites on mechanical performance and least environmental impact. Many promising products have been demonstrated, but mostly for small production volumes in niche markets. Application for medium to large production volumes is to be developed for high production parameters.
The application of glass-fibre reinforced composites (GFRC) is widely used in lightweight construction in various fields of application, for example in the aerospace industry. However, GFRCs still have some serious environmental disadvantages: production of the raw material of the glass fiber in countries with low energy costs such as China, as well as long transportation distances for the raw materials to Europe. In addition, a lot of energy is required for the production of glass, which is associated with high carbon dioxide (CO2) emissions. To produce just one ton of glass fibers, 30 gigajoules (GJ), or 8300 kilowatt-hours (kWh), are required, and 4800 kilograms (kg) of CO2 are generated [MPB11].
Natural fibers, such as flax fibers, have the potential to replace glass fibers because of their mechanical properties, thus reducing the environmental impact caused by the use of GFRC. In particular, the CO2 footprint of natural fibers is much lower than for glass fibers. For the production of one ton of natural fibers, only 5 GJ are needed and 800 kg CO2 is generated [MPB11]. The saving compared to glass is therefore 83 percent. Furthermore, high-quality flax fibers that are suitable for use in structural components are produced in France, Belgium and the Netherlands, [BS13] while glass fibers mostly are produced in distant countries, especially in China. As a result, the environmental load can be considerably reduced by the shorter transport of the fibers. The use of natural fiber reinforced composites (NFRC) can further reduce the environmental impact because NFRCs are suitable for cascade use due to their high self-energy [BS13] and, unlike GFRC, do not have to be deposited after their end of use. Rather, waste residues from the production of semi-finished products can be composted as required, since the fibers are a natural product. NFRC products can be melted again when a thermoplastic matrix is used and thus the matrix can be separated from the natural fiber. The natural fiber can be reused in injection mouldings. The thermoplastic matrix can be reused in various applications. Furthermore, in the case of components with a thermoset matrix — also in the case of a thermoplastic matrix — the self-sufficiency can be fully recovered by cascade use. Therefore, an environmentally damaging landfill is not necessary for NFRC.
However, there are still challenges for all advantages: In composite materials, reinforcing fibers must always be aligned in load direction in order to be able to exploit the maximum mechanical properties of the fibers in the composite. This is not a problem with glass fibers, since they are produced synthetically and are virtually endless. The further processing of glass fibers to fabrics, for example by weaving, can be carried out directly in the following process step. Natural fibers, on the other hand, are basically staple fibers, that is to say fibers having a defined length of 30 to 100 millimeters. The prior art is to form these staple fibers into a sliver and to provide sufficient strength for the further processing like weaving by applying a twist to the drawn fiber package. However, this twist in the yarn causes the fibers in the composite component to no longer be in load direction. Thus, their strength cannot be fully utilized. Therefore, natural fibers have hitherto only been used in non-structural components with no loads and in non-visible areas, such as, for car inner door paneling.
The main problem for both traditional and bio-based composites is the relatively high cost of average 30 euros per kilogram as the result of a still labour-intensive manufacturing process. As a consequence, the market for bio-based composites is hardly opened yet, leaving many promising sustainable technologies unexploited. But the market for this new material is growing at a rate of 100,000 tons per year in the industry’s highest-selling NFRC market. In 2005, the NFRC volume in the automotive industry was only 30,000 tons [KOG+06].
When using NFRC, same mechanical properties are achieved as with GFRC with an additional higher damping capacity. The higher damping capacity of flax fibers with the same mechanical properties allows a very wide range of application of NFRC in the automobile and for sports equipment such as hockey sticks and alpine skis. Carbon and glass fiber composites are only limited suitable for this, known to have partly better mechanical properties are flax fibers.
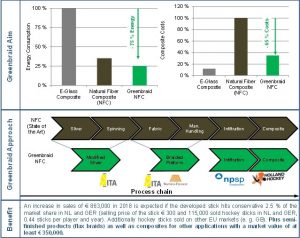
The aim of the project is to reduce the energy consumption of fiber reinforced composite products by 75 percent at same process costs using the example of a hockey stick (See Figure 1). The approach is the elimination of process steps during the spinning process and increasing the level of automation during the preform production. A field hockey stick is manufactured as a demonstrator, which, due to its complex, curved geometry, serves as a platform technology for further applications. The demonstrator is compared with a glass fiber hockey stick. The research will provide knowledge basis for future transfer to applications like windmill blades, pressure vessels or automotive scooters. With the help of this project the industry will be able to produce bio composites for high performance materials.
Approach
The novel flax fiber hockey sticks consist of flax braids formed around a foam core, impregnated by a resin under vacuum pressure using an elastic tubular foil. Before braiding the flax fibers are spun to yarn with low/no twist using a special spinning technology. The approach is new and relies heavily on dedicated designed braids.
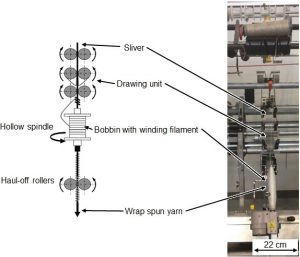
Wrap Spinning Technology
The special method of the wrap spinning technology is used, in which a winding filament is laid around the drawn sliver of flax fibers. The core remains untwisted and is solidified solely by the winding filament (See Figure 2). Afterwards the wrap spun yarn can be braided and resinated with a low-viscosity epoxy resin.
Through this approach, it is for the first time possible to use cost-efficient, environmentally-friendly natural fibers as reinforcing fibers in composite materials for structural applications and thus to substitute glass fibers. Natural fibers, which have a limited length, can thereby be further processed by means of conventional surface forming methods and can be introduced into the composite component in a completely aligned manner.

Figure 3 shows a wrap spun yarn with flax fibers in the core and a nylon 6,6 filament. The low twist flax roving with the count of 400 tex was purchased from France-based Safilin. The draw ratio was set 0 due to the already very fine roving. The winding filament was wrapped around the roving with 100 windings per meter. The draw-off speed was 20 meters per minute but can be increased drastically if needed with no yarn breaks occurring.
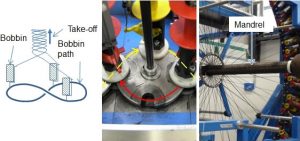
Braiding Process
Net-shaped textile structures, known as preforms within the composites industry, can be produced in a radial braiding process. In this process, fibers like carbon or glass can be used to over-braid a net-shaped mandrel (See Figure 4).
This process can be used to produce curved hollow structures for example for car components or sport articles like hockey sticks. Radial braiding is already used in mass production by BMW Group, Germany. Some components of the i- and M-series cars are integrated in the body using glass and carbon fibers.
The novelty in the frame of this research is the ability to process also bio-based fibers like flax or linen due the innovative process of fiber wrap spinning technology and optimizing the machine set-up.
Optimizing the process parameters for braiding is determined by two conflicting criteria. The first is by the braiding process in order not to break the yarns; the other resides from the design of the product. As braiding is a highly automated process involving an extensive preparation of the machine, breakage would increase costs for recovering the process. However, first results of braiding of flax fibers are shown in Figure 5.
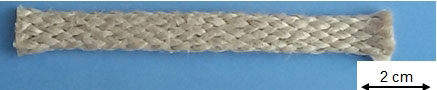
This result shows the process ability by setting the machine properly. But there are still some broken filaments that will lead to decreased mechanical properties. Therefore, ITA has developed a concept featuring a fiber carrier bobbin that is designed to reduce fiber damage and to set even the fiber tension during the process to adjust it to the curved hockey stick. This will also lead to better fiber distribution on curved areas of the product. The concept of the automated carrier will be patented and, therefore, not discussed in detail here.
Outlook
Next steps in this research are to adjust the fiber architecture to achieve mechanical properties comparable to glass fiber composites and to evaluate mechanical properties. The approach is to braid the modified flax on a mandrel and to cut out test specimens off the hose. The test specimens will be consolidated and tested. For the production of the test specimens the radial braider Herzog RF 144-100/1 is used. Bending and impact behavior will be analyzed. Furthermore, the amount of fiber damage needs to be identified and compared with the damage using of the new design of the bobbin. For this purpose, at least 10 bobbins will be built and installed in the machine.
References
[BS13] Brückner, T.; Steger, J.: Quantitative und qualitative Bedarfsanalyse für Naturfasern und Optionen zur regionalen Sicherung der Rohstoffbereitstellung in Deutschland. Final report. Funding organisation Fachagentur für nachwachsende Rohstoffe (FNR), Förderkennzeichen 22034311. SachsenLeinen GmbH, Waldenburg, 2013
[MPB11] Morasch, A.; Prievitzer, J.; Baier, H.: Zur ganzheitlichen Bewertung von Werkstoffen am Beispiel von naturfaserverstärkten und glasfaserverstärkten Kunststoffen. Presentation. Lehrstuhl für Leichtbau (LLB), Technische Universität München, München, 29.09.2011
[CHE11] Cherif, Ch.: Textile Werkstoffe für den Leichtbau. Berlin, Heidelberg: Springer- Verl., 2011 ISBN: 978-3-642-17991-4
[KOG+06] Karus, M.; Ortmann, S.; Gahle, C.; Pendarovski, C.: Einsatz von Naturfasern in Verbundwerkstoffen für die Automobilproduktion in Deutschland von 1999 bis 29005. Nova-Institut. Hürth, November 2006. http://www.nova-institut.de/pdf/06-11NF-VerbundAutoD.pdf, accessed on January 25,2017
Editor’s Notes: Dipl.-Ing. Marie-Isabel Popzyk; Viktor Reimer, M.Sc.; and Dr. Thomas Gries, professor and head; with the Institut für Textiltechnik, the textile machinery and processes research arm of RWTH Aachen University, Germany, contributed to this article. Grateful acknowledgement goes to the research association Eurostars of the research project “GreenBraid”. The author would also like to thank the project partners NPSP and Holland Hockey both based in The Netherlands, and Germany-based Barthels-Feldhoff.
June 2017